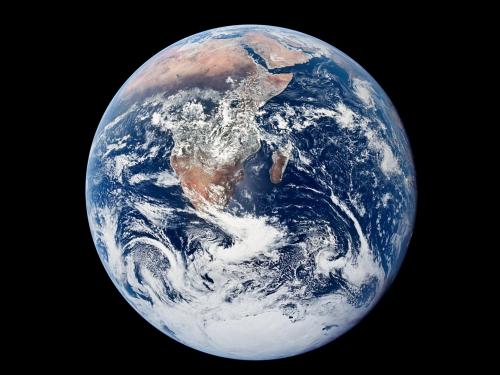
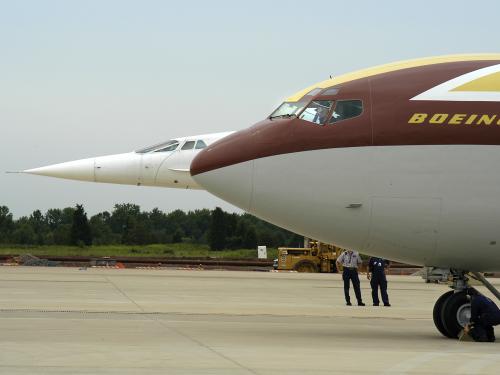
Dec 18, 2024
By Mark Piesing
A global early-warning system strives to keep aircraft safe from volcanic eruptions.
At the start of a scheduled flight from London to Auckland, New Zealand, British Airways Flight 009 thundered down the runway at London Heathrow. The takeoff, which occurred in the early evening on June 23, 1982, was uneventful. A few hours later, however, passenger Charles Capewell was writing a farewell note: “Ma In Trouble Plane going down will do best for the boys. We love you.”
On the third leg of the transoceanic flight, the three-year-old Boeing 747-200 City of Edinburgh was flying at 37,000 feet when the crew observed something strange outside the cockpit. What they saw had the “appearance of millions of machine gun bullets or sparks,” according to Betty Tootell, eyewitness and author of the 1985 book All Four Engines Have Failed. Onlookers assumed it was a weather phenomenon known as St. Elmo’s fire, which creates a luminous electrical discharge.
Suddenly, the 747’s no. 4 engine flamed out. This wasn’t a disaster, however, because a 747 can fly on three engines. But it can’t fly on zero engines. Less than a minute later, no. 2 shut down, quickly followed by no. 1 and no. 3.
In only a few minutes, an $83 million jetliner had turned into an 833,000-pound glider—and no one on board knew why.
Captain Eric Moody made a typically British announcement to the passengers: “Ladies and gentlemen, this is your captain speaking. We have a small problem. All four engines have stopped. We are doing our damnedest to get it under control. I trust you are not in too much distress.”
Unbeknownst to the flight crew, BA 009 was 93 miles downwind of the eruption of the Galunggung volcano in West Java, Indonesia, and the airliner had flown into an enormous ash cloud.
The eruption of Mount Augustine in 2006 lasted for several months, significantly disrupting commercial airline flights in western Alaska.
Earth is a geologically active planet, with a core nearly the temperature of the surface of the sun. More than 80 percent of the planet’s surface is of volcanic origin. According to the Global Volcanism Program at the Smithsonian’s National Museum of Natural History, over 1,100 volcanoes have erupted since the last ice age, and out of these, more than 500 are still active.
The aptly named “ring of fire,” which includes 127 active volcanoes, is roughly 2,500 miles long and up to about 310 miles wide, encircling most of the Pacific Ocean. The United States has around 170 historically active volcanoes, 55 of which are in Alaska, including five that are categorized as high threat.
Volcanic eruptions pit some of the most expensive and sophisticated technology on Earth against one of the planet’s most powerful natural forces. The amount of gas and highly abrasive pulverized rock debris (ash) that is ejected into the atmosphere during an eruption can cause havoc to modern jet aircraft. And the 10-fold increase in air transport since 1970 means that ash and airliners occupy the same space more often.
One study found that there have been nearly as many volcanic encounters with aircraft in the last 14 years as in the previous 56 years, with 113 incidents since 2010. All but four of these incidents were related to eruptions of volcanoes in Iceland—Eyjafjallajökull in 2010 and 2017 and Grimsvötn in 2011.
“Has exposure to volcanic activity gone up?” asks David J. Schneider, a research geophysicist at the U.S. Geological Survey’s Alaska Volcano Observatory who oversees a project to mitigate the hazardous impact of volcanic ash. “Absolutely, yes. The level of volcanic activity has remained more or less constant, but there are more people flying nowadays, and that will absolutely increase exposure to it.”
In the more than 100 incidents over the decades, there were two terrifying stories that alerted the aviation world to how imperiling volcanic ash can be.
The first was the aforementioned BA 009. After descending to an altitude of 13,500 feet, the pilots made one last attempt to get the engines going. Suddenly, no. 4 burst to life. Eighty seconds later, engine no. 3 restarted, and then 1 and 2.
“Jakarta, we’re back in business,” radioed senior flight officer Roger Greaves. “All four engines running.” Moments later, engine no. 2 had to be shut down. But the pilots were now confident they could safely land at Jakarta’s Halim Perdanakusuma International Airport.
Abrasive volcanic ash can cause extensive damage to forward-facing surfaces of an airplane, including the cockpit windows, landing-light covers, and engine nose cowls.
The second ash encounter occurred seven years later, in the skies above Alaska. Mount Redoubt has many names. But whether in English, Russian, or the local language Dena’ina, they all mean roughly the same thing, a “fortified place”—and it’s easy to see why. At 10,197 feet, Redoubt is the highest mountain in the Aleutian Range. It has been an active volcano for more than a millennium. In December 1989, it erupted for the third time in the 20th century, spewing volcanic ash to an altitude of 45,000 feet. KLM Flight 867, carrying 231 passengers, was caught directly in the plume.
Like BA 009, the KLM jumbo jet lost power in all four engines, and again the pilots struggled to control the aircraft as it plummeted from 27,900 feet toward the sea. At 13,300 feet, the crew managed
to restart the engines, and they landed safely in Anchorage.
The damage to the aircraft—its engines, avionics, and airframe—was severe. All four engines needed to be replaced, and the total repair bill was estimated at $80 million, almost as expensive as a new 747 at the time.
Mount Redoubt had not yet finished with commercial aviation. Two days later, another two jet airliners encountered the same ash plume. But this time, it was over west Texas. The ash had traveled more than 3,300 miles in some 55 hours.
Volcanoes can make their presence felt far beyond their locations. Another reminder of this inconvenient fact came two years later when, on June 15, 1991, Mount Pinatubo in the Philippines let loose. Three days later, the ash cloud had been blown 5,000 miles across the Indian Ocean to reach the coast of Africa. Around 20 airliners encountered the ash—and nearly all of them did so more than 600 miles from the volcano.
In 2015, NASA researchers studied the effects of volcanic eruptions on aircraft by pumping ash directly into the core of an engine.
The accumulating incidents became a catalyst for change. Following the flameout of British Airways Flight 009 in 1982, the International Civil Aviation Organization (ICAO) created the International Airways Volcano Watch, which later became a volcano monitoring program. This, in turn, led to the establishment of nine global Volcanic Ash Advisory Centers. They use seismic and other ground-based data collected by volcanologists stationed at volcano observatories and combine it with remote-sensing data to enable aircraft to reroute their flight paths.
A scanning electron microscope image of volcanic ash reveals the sharp silica fragments that can erode compressor airfoils.
The Smithsonian’s Global Volcanism Program also provides information to ICAO. The program maintains a database documenting volcanoes that have erupted on Earth in the past 12,000 years and up through the present. During the early stages of an eruption anywhere in the world, the Smithsonian program acts as a clearinghouse of reports, data, and imagery.
Real-time reporting is crucial. Sometimes even personal contacts can make a difference. In 2009, for instance, a sudden eruption on a remote island at Sarychev Peak in Russia generated a plume that later spread over a large area of the North Pacific and disrupted international air traffic. Initial details of the eruption remained vague until a Russian team aboard a trawler visited the remote island. A volunteer with the Smithsonian program who spoke Russian contacted the team leader, an old colleague, and persuaded him to contribute a report from the scene.
For a pilot, the first signs of flying into an ash cloud can include sudden darkness, fluctuating airspeed, and landing lights that cast sharp, distinct shadows. Inside the cabin, there might be dust and an acrid burning smell. Then, a bright, white-orange glow appears in the engine inlets.
At that point, the volcanic ash has already started to foul the engines. Volcanic ash is far more abrasive than dust and sand. It’s made of sharp silica fragments that have a low melting temperature. Due to the explosive nature of volcanoes, the fragments can be propelled high into the atmosphere.
A plume of ash from Italy’s Mount Etna rises above a pair of U.S. Navy Boeing P-8A Poseidons assigned to the “Grey Knights” of Patrol Squadron 46.
When an aircraft flies through an ash cloud, the ash is drawn through the engine fan and into the compressors, eroding the compressor airfoils. The ash continues its path into the combustor, where the high temperatures of the burning air-fuel mixture cause the silica to melt; it then flows into the turbine section of the engine. The molten glass sticks to vital engine components building up as a deposit on combustor surfaces and the high pressure turbine inlet airfoils.
The ash doesn’t need to completely gum up an engine to cause flameouts like those experienced by BA 009 and KLM Flight 867. “You just need to block its hot section by between five, maybe 10, even 20 percent to reduce the flow enough to cause the compressors not to work well,” says Rory Clarkson, an associate fellow on the engine environmental protection team at Rolls-Royce Civil Aerospace.
That’s not all. The forward-facing surfaces of an airplane—whether plastic, metal, or glass—can be pitted while flying through an ash cloud. Such harsh abrasion can irreparably harm the cockpit and forward cabin windows, landing-light covers, engine nose cowls, thrust reversers, and pitot tubes.
If ash penetrates sensitive aircraft electronics, it can interfere with navigation and other onboard systems. The flight crew could lose the ability to communicate with passengers and flight attendants in the cabin. What’s more, electrical disturbances within the ash cloud might suppress the ability to transmit a distress call.
Researchers from the Hawaiian Volcano Observatory take laser rangefinder measurements of a lava flow. Volcanologists worldwide share their data with nine global Volcanic Ash Advisory Centers.
An eruption can also affect airport operations. Ash falling on and near airports can make approach and departure particularly hazardous, as well as hindering operations on the ground. Ash on runways makes braking tricky, and wet ash is extremely slippery. And the ash-blasted windscreens of an airliner can make landing and taxiing perilous.
The volcanoes of Indonesia, the Philippines, and the rest of the Ring of Fire tend to erupt explosively, and many produce a drifting ash cloud that can be hazardous for days afterward. The ash cloud from the 2010 Eyjafjallajökull eruption, for example, reached 30,000 feet and spread across the United Kingdom and the European continent.
Alaska has more than 100 volcanoes, including Mount Cleveland (top) and Mount Carlisle (foreground).
The Vikings named Iceland the “land of snow.” But the Nordic country sits above a fracture in the Earth’s crust, which permits magma to rise to the surface and erupt as lava and ash. Iceland experiences an eruption every five years on average.
Around three-quarters of the eruptions produce ash, but for many years the airline industry encountered only limited disruption. Its luck ran out in 2010.
A satellite image shows a 250-mile-long ash plume emerging from the Eyjafjallajoekull volcano in Iceland. The 2010 eruption grounded 100,000 commercial flights.
Eyjafjallajökull doesn’t resemble what people think of as a typical volcano. Rather than a cone shape, it looks like a shield placed on the ground and covered by a 25-square-mile ice cap. Its eruption on March 20 was nothing out of the ordinary for Iceland’s volcanoes, and for the first couple of weeks, lava oozed from its ice-free side, and the ash plume it produced was not problematic.
Three weeks later, though, on April 14, molten rock began to flow from its crater, and once meltwater from the ice interacted with lava, the eruptions became violent. The spectacular plume of ash that Eyjafjallajökull expelled soon reached mainland Europe and caused, according to Science, the “largest disruption of the continent’s air traffic since World War II.”
Last August, a fissure erupted at the Sundhnúksgígar volcano on the Reykjanes Peninsula, just 12 miles from Iceland’s main international airport.
Regulators ordered a shutdown of much of Europe’s air traffic, which lasted for seven days. More than 100,000 flights were cancelled, affecting 10 million passengers. This in turn had an impact on tourism, freight, and international trade—all still recovering from the 2008 economic crisis. The cumulative economic impact was later estimated to be $5 billion.
“When Eyjafjallajökull erupted in 2010, the airline industry standard was essentially zero tolerance of ash,” says Jim Zimbelman, senior geologist emeritus at the National Air and Space Museum’s Center for Earth and Planetary Studies. “That is, if there was any indication of ash at any level, you couldn’t fly. And this zero-tolerance policy is what caused Europe to shut down.
Drones enable researchers to gather samples from volcanoes that are otherwise difficult and dangerous to reach.
“Well, someone during that timeframe realized, look, that’s not realistic,” he says. “We’ve got to find out what is more appropriate for actual engines, and they came up with less than two milligrams of ash per cubic meter. It doesn’t sound like a lot, but it means that the engines can still operate if it’s less than that. They may be damaged, but you won’t have total flameouts like over Indonesia and Alaska, and that minimum allowed airspaces to reopen.”
While the new safety standard got everyone back in the air, questions persisted about the accuracy of methods used to quantify the threat. After the Eyjafjallajökull incident, European aviation authorities endorsed the creation of ash concentration charts that sought to help aircraft navigate a safe passage through high-altitude ash plumes. “But the effort was not fully successful,” the U.S. Geological Survey said in 2012. “The ash-dispersion computer models used to forecast ash concentrations downwind of an erupting volcano were found to have large uncertainties, as much as plus or minus 10-fold.”
There was also confusion over how flight crews should assess the risks. Two milligrams of ash per cubic meter—the density of ash that had been chosen as the threshold for aircraft safety—represented the concentration of ash that could be seen with the naked eye. But an international task force later clarified that aircraft needed to rely on more than what was visible to flight crews—especially when previous ash clouds went undetected by flight crews because the ash had been blocked by ice and water clouds or the encounter happened during the dark of night.
A DC-10 sits on its tail, weighed down by wet ash from the June 15, 1991 eruption of Mount Pinatubo in the Philippines.
In 2013, the International Civil Aviation Organization introduced the concept of “discernible ash,” which is “volcanic ash detected by defined impacts on/in aircraft or by agreed in-situ and/or remote-sensing techniques.” Put another way, flight crews would assess the risk based on what they saw and whatever data could be provided by scientific instruments, which have proliferated in the 15 years since Iceland’s Eyjafjallajökull shut down the skies. Countries improved ash measurements, for example, by installing additional ground-based LIDAR stations. LIDAR, which is short for light detection and ranging, shoots bursts of light from a laser into the sky to acquire information on the physical properties of the atmosphere. LIDAR stations can detect particles as small as 0.1 micrometers in size.
Data and imagery provided by the European Space Agency’s Sentinel satellites, first launched in 2014, have also enhanced volcanic ash forecasts, as has the United States’ Suomi National Polar-orbiting Partnership weather satellite, which was launched in 2011.
“That is all a definite improvement from the 1989 situation in Alaska, when none of that really was going on,” says Schneider. Still, even with improvements in remote sensing technology, the U.S. Geological Survey acknowledged that a key issue remained: “whether aircraft can fly through diluted ash clouds safely...and, if so, for how long.”
Data provided by the aviation industry would prove crucial in addressing the question. Notably, between 2010 and 2016, Rolls-Royce, the world’s second-largest manufacturer of aircraft engines, conducted tests on engine susceptibility to volcanic ash.
NASA’s Landsat 5 satellite captured an image of Mount Redoubt erupting in 2009. Twenty years earlier, a 747-400 temporarily lost all four of its engines after encountering an ash cloud from the same volcano.
Their research confirmed that a threat assessment should be based not only on the concentration of ash, but also on the duration of exposure—the cumulative dose of volcanic ash. More specifically, the engineers found that the company’s Trent and RB211 engines could operate safely for one hour amid ash concentrations as high as four milligrams per cubic meter, or for two hours flying through two milligrams of ash per cubic meter.
In 2022, ICAO announced that, by 2025, all Volcanic Ash Advisory Centers would provide airline operators with high-resolution volcanic ash forecasts every three hours in the event of an eruption. The forecasts, which are called quantitative volcanic ash (QVA) data, present a range of ash concentration measurements—ranging from “very low” (less than two milligrams per cubic meter) to “very high” (more than two milligrams per cubic meter)—enabling flight crews to determine how best to chart their course, taking into account flight duration through an ash cloud as well as its density. “QVA information offers operators the opportunity to move away from traditional discernible-visible ash criteria and instead use certified engine susceptibility for flight route planning and inflight replanning,” said a statement from ICAO.
Like weather forecasting, volcanic ash assessment is a perpetual work-in-progress as researchers gain access to more data and higher computing power. A scientific team at the University of Cambridge, for instance, is using video analysis to study the characteristics of an ash cloud—such as its shape and speed—at various time intervals during an eruption. While studying footage of the 2010 Eyjafjallajökull eruption, researchers were surprised to discover that, at one point, the rate of ash flow dropped by about half. Such data can help researchers better measure and model how much ash goes up into the atmosphere, how high the ash cloud will go, how long the plume will stay buoyant, and how quickly the ash will start falling to the ground.
Other scientists are working on improving the monitoring system. “Each member state of ICAO, if they’ve got volcanoes, they’re supposed to report on volcanoes, but there is an uneven distribution of capability to do that,” says Schneider. “The countries not doing it tend to be in places where there’s not a lot of access to resources to be part of it, but that’s something we’ve been working on slowly over the years with our volcanological colleagues through building personal relationships.”
Some researchers think that the next step beyond these initiatives will be to create a worldwide warning system for volcanic hazards of all kinds, and not just ash clouds for aviation.
“Volcanoes cause a lot of direct deaths, whether from pyroclastic flows, lahars, volcanic gases, and of course, a huge eruption itself,” says Andrew Tupper, founder of Natural Hazards Consulting. “There are all kinds of reasons why we want to monitor volcanoes, and if we manage to successfully do that for all hazards, then we’re also helping aviation as well.”
Mark Piesing is an aviation journalist and frequent contributor to Air & Space Quarterly. He is the author of N-4 Down: The Hunt for the Arctic Airship Italia (Custom House, 2021).
This article, originally titled “Plumes and Planes (Don’t Mix),” is from the Winter 2025 issue of Air & Space Quarterly, the National Air and Space Museum's signature magazine that explores topics in aviation and space, from the earliest moments of flight to today. Explore the full issue.
Want to receive ad-free hard copies of Air & Space Quarterly? Join the Museum's National Air and Space Society to subscribe.
We rely on the generous support of donors, sponsors, members, and other benefactors to share the history and impact of aviation and spaceflight, educate the public, and inspire future generations. With your help, we can continue to preserve and safeguard the world’s most comprehensive collection of artifacts representing the great achievements of flight and space exploration.
We rely on the generous support of donors, sponsors, members, and other benefactors to share the history and impact of aviation and spaceflight, educate the public, and inspire future generations. With your help, we can continue to preserve and safeguard the world’s most comprehensive collection of artifacts representing the great achievements of flight and space exploration.